Positron emission tomography
1997/03/01 Campillo Robles, Jose Miguel - Fisika SailaZientzia eta Teknologia Fakultatea (EHU) | Urzelai Pinedo, Ainhoa Iturria: Elhuyar aldizkaria
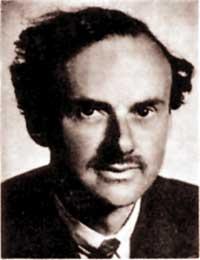
To do this, we will explain a nuclear technique used in medicine for research and study: Positron emission tomography (PIT), popularly known as Positron Emission Tomography. This technique began to develop in medicine in 1953, in order to observe the functioning of the body through images. The physical basis of the technique lies in the physics of positron, so we will begin to study the physics of positron, to then clarify the details of the technique.
What is positron?
Positron is the antiparticle of the electron. Being an antiparticle, it contains charge and energy against the corresponding particle. Therefore, by definition having a negative charge, positron has a positive charge. As for the mass, the positron has the same mass at rest as the electron. Table 1 summarizes the characteristics of the electron and positron pass.
Discovery of positron
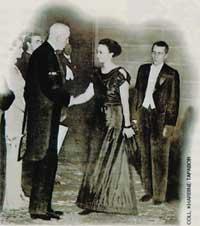
Paul Adrien Maurice Dirac (1902-1984) made the theoretical discovery of positron in 1928. At that time, Dirac investigated the relativistic quantum equation of the electron, obtaining between its results two solutions for the value of energy, one positive and one negative:
where m or p are the resting mass and the linear moment of the electron and c the speed of light. In the beginning he remakes the calculations made, with the suspicion that they were ill-done. Finally he realized that they were well and then the positive sign solution was assigned to the electron, but it was not known what the negative sign corresponded to. Later, scientists realized that this resolution was of a new particle and the new particle found was called positron. The theory of holes is what Dirac developed to explain the electron/positron system. To know the resting energy of these two particles, the above equation must be used, obtaining the values shown in Table 1.
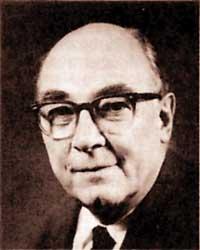
Carl David Anderson (1905-1991) made the first experimental observation in 1932. This observation took place in the Wilson fog chamber, located in a magnetic field. Anderson wanted to analyze the interaction between cosmic rays and matter through this camera. However, during the observation of the positron, he did not know the works of Dirac. So he didn't know what that particle he found. Until 1939, it was not demonstrated that the properties of the new experimentally observed particle were the same as predicated by Dirac's theory. Once clarified, Anderson named the positron. This discovery earned him the Nobel Prize in Physics in 1936, being the first positron antiparticle observed.
In 1934 Irene Curie (1897-1956) and her husband, Jean Frédéric Joliot (1900-1958), found their first positron in laboratory conditions. The couples bombarded the light cores with particles a and then observed the b + disintegration in the samples. In particular, after the collision with particles Al, B and Mg, a, the artificial radioactive elements P, N and Si were created. Thus, by the discovery of artificial radioactivity, these scientists obtained the Nobel Prize in Chemistry in 1935.
Electron - e - | Positron - e+ | |
Load (C) | -1.60219x10 -19 | + 1.60219x10 -19 |
Power (keV) | + 511 | - 511 |
Mass (g) | Information | Information |
Spin | 1 of 2 | 1 of 2 |
How do positrons occur?
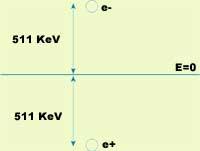
Our Universe, at least this known piece, consists mainly of matters. Therefore, since they are antimateria positrones, we cannot find them anywhere. That being so, how do they appear? Positrons can be produced by two ways, one of the high-energy radiation by forming pairs e – /e + and another by disintegrating b +. To advance our explanations we will analyze these two ways.
The source of positron that we will first analyze is the formation of particle/antiparticle pairs. These couples come from a high energy photon. To do this, the energy of the photon must be at least greater than the energy of the pair at rest. This statement is expressed mathematically by the following formula.
where "Planck constant, photon frequency n, m or particle resting mass (antipart) and c the speed of light.
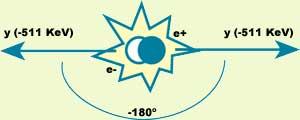
The second source of positrons consists of disintegration b +. The positrons used in the PIT technique are generated through this process. b + we will use an example to see what disintegration is. Suppose we have oxygen atoms. The nucleus of an oxygen atom has 8 protons and 10 neutrons (18O). If we bomb the atoms we have by protons (p+), the core of an oxygen can take a proton. If this happens, the atom will be another element, the fluoro, which will release a neutral (n).
18O + p + 18F + n
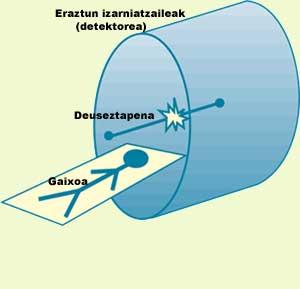
This fluoro isotope has 9 protons and 9 neutrons. However, for a fluoro atom to be stable it must have 10 neutrons, i.e. 19 F. 18 The core of F has one less neutron, so it is not stable. Then, to reach stability, a nucleus proton will become neutron and in that process a prositroi and neutrino (n) will be emitted, as we can see in the following formula.
p + n + e + n
In this way, the isotope of fluoro is transformed back into oxygen. In this example protons have been used for bombardment, but other types of particles, such as a particles or deuterium, can be used.
How do they disappear?
When positron is generated by b + disintegration it has a great kinetic energy (around MeV). The positron is accompanied by a neutrino. This has very little mass (if it has mass, as it is not currently known) and has no load. Therefore, the interaction between neutrino and matter is very small and we do not take it into account.
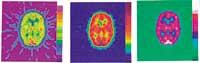
Its kinetic energy when positron is generated is lost due to the process we call thermalization, until it stops. The length of the average travel you make depends mainly on the material you are in. For example, in water it is a few millimeters; as 75% of people's body weight is water, the average travel length of a positron is also millimeter in the body. Another factor that influences the length of the average travel is the nature of the isotope used. 18 In the case of isotope F, the average trajectory is 1.2 mm and in the case of 82 Rb is 12.4 mm.
After thermalization, the positron is ready for removal. Elimination occurs when the positron meets an electron. Then, Dirac's hole theory explains that the electron already lowers positron to the energy level, releasing all the energy from the mass system. In particular, after the destruction of the electron and positron, two photons appear dragging all the energy. In this process it is also necessary to preserve kinetic energy, so in the energy of the two photons there will be the two previous contributions. If the electron is supposed to stand as an approximation, the photons are 511 keV, i.e. they are gamma photons (g).
In elimination, in addition to energy, the linear moment must be preserved. If we continue to assume that the electron is standing, the linear moment of the system of the two photons generated after disposal will also be null. To make this possible, the photons will move in the same direction and in the opposite direction (see figure 2).
So far we have stopped the electron, but this is not entirely true. Before removal occurs, the electron has kinetic energy and its effect is that the angle between photon trajectories is not exactly 180° and its energy is slightly removed from the ideal value 511 keV.
What is the PIT used for?
In medicine we are often interested in knowing where the substances go inside the body, how they reach the organs or how long it takes to arrive or leave. In many cases it is not easy to obtain this information. The PIT we analyzed here was developed to know all this technique. The goal of this tomography is to see how the chemical and physiological processes that occur within our body work. That is, with PIT you can study metabolism or many different body functions. This technique has been applied in many fields of medicine, such as neurology or cardiology.
What is the PIT?
To begin with, we mark the substance we want to follow through the body, fixing the isotopes emitting positron to this substance. The markers used in the PIT technique are the molecules present in the body. Therefore, the physical-chemical behavior of the marked substances is equal to that of the unmarked substances.
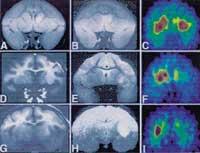
The markers are selected based on the part and function of the body we want to study and the isotope placed in them. For example, if the isotope 15 O (2.2 minutes of half-life) adheres to water molecules, it can be used to see the transport of cerebral blood, but if used in oxygen molecules it can be analyzed the use of oxygen in metabolism; similarly, the isotope 18 F (half life 110 minutes) is used glued to fluorodeoxicosa to see regional brain metabolism.
When the marked substance is inside the patient, it is inserted into starry rings. The function of these rings is photon detection. Photon trajectories are supposed to be 180°. Therefore, analyzing the places of arrival of the two photons and the time difference they have had upon arrival, you can know where the removal of electrons/positrons has occurred. As removal occurs “near” the emitting isotope, we will have information about the location of the isotope, as can be seen in Figure 3.
As the isotope moves inside the body, the photons will be detected in different places, allowing us to follow the movement of the substance that interests us, observing how far it reaches.
The data obtained are properly processed and, finally, an interpretation of the results is made using software prepared for this purpose. Figure 4 shows the importance of data manipulation. In the figure above, the same data is manipulated in different ways to obtain three apparently different images.
Advantages and problems
The PIT technique has many advantages that other procedures do not have. Most diseases attack the body biochemically before damaging it. Therefore it is convenient to study the chemistry of the body. The PIT technique can perform quantitative measurements in vivo of the functional processes of the body. This way you can complete the information provided by other techniques. For example, magnetic resonance imaging produces more accurate images and is sometimes used along with PIT imaging (see figure 6)
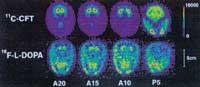
Another major advantage is that the isotopes used are the elements in the body. And it is that substances that can be rare for the body are not used and that, of course, to the benefit of our body. In addition, due to the high sensitivity of the technique, these compounds are used in very small amounts (less than the nanomolar), so the patient's metabolism does not vary.
On the other hand, the short half-life of the isotopes makes the research fast and the patient receives small doses of radiation. This dose of radiation is similar to that which should be supported in the manufacture of two x-ray plates in the chest.
Along with the advantages, we must mention the problems. One of them is precision. As mentioned above, the angle between photon paths is not exactly 180°. This reduces accuracy, but however, the biggest generator error is the positron path before deletion. Through the technique we know where the elimination occurs, but the isotope is a few millimeters away.
The last disadvantage we will mention is not directly related to science, but it can be important. We must talk about the economic problem. This technique is very expensive, as isotopes are formed by a cyclotron. The price of the cyclotron is very low, so the PIT technique is not widespread. For example, in Euskal Herria it still does not apply.
Finally, it should be noted that all techniques that use radiation are dangerous, so the PIT technique is no exception. Only when strictly necessary.
More information http://www.topo.ucl.ac.be Related links: Iñigo Alonso, “Basic particles”, XXI UEU. Talk at the Summer Courses given in 1993 in Pamplona (unpublished) UEU Department of Physics, “Brief history of Physics”, Department of Heda 9, Basque Summer University, Pamplona, 1990. |
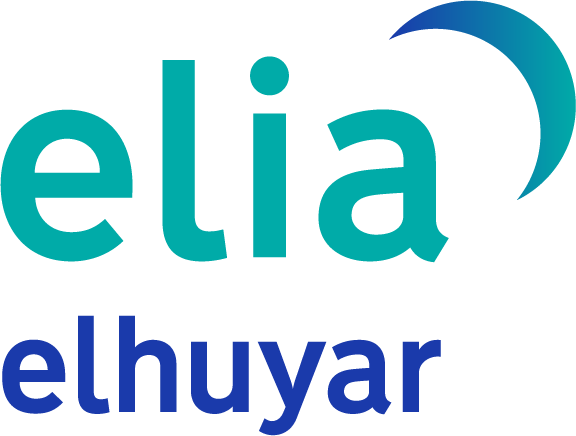
Gai honi buruzko eduki gehiago
Elhuyarrek garatutako teknologia